Optical Coherence Tomography (OCT) is a microscopy and ophthalmoscopy technology that has changed the paradigms for clinical analysis of retinal and eye diseases [1]. First developed and implemented in the form of a Scanning Laser Ophthalmoscope (SLO) in the 1980s, OCT has opened a new window for super-resolution imaging in microscopy, allowing for the analysis of the different layers that form the retina and the diagnosis and treatment of glaucoma and other macular diseases.
The main advantages of the OCT technique are related to the non-invasiveness, leading to cross-sectional biopsies of the biological tissues and the high axial resolution of the technique, which makes it possible to screen layers of the retina that are between 5 and 20 micrometres deep.
General working Principle of OCT
Optical Coherence Tomography uses a low-coherence interferometer system to compare the delay between the reflected light that passed through the sample, and the reference light beam, whose length is already known. According to that delay in time, quantifications about the depth of the tissues are performed. This principle is referred to as Temporal Coherence. In other words, near-infrared light from an optical source reaches an interferometer that splits the light path into two arms. One of the beams (reference beam) will go directly towards the detector; whereas the other beam (probe beam) will reach the eye, travel towards the retina and will be reflected back, to exit the eye [1]. The two reflected beams are combined at the output of the interferometer. OCT requires low temporal coherence light sources in order to match the phase of the reference and probe beam over a time travel interval called coherence length.
A schematic representation of an interferometer for time-domain OCT is shown in Figure 1:
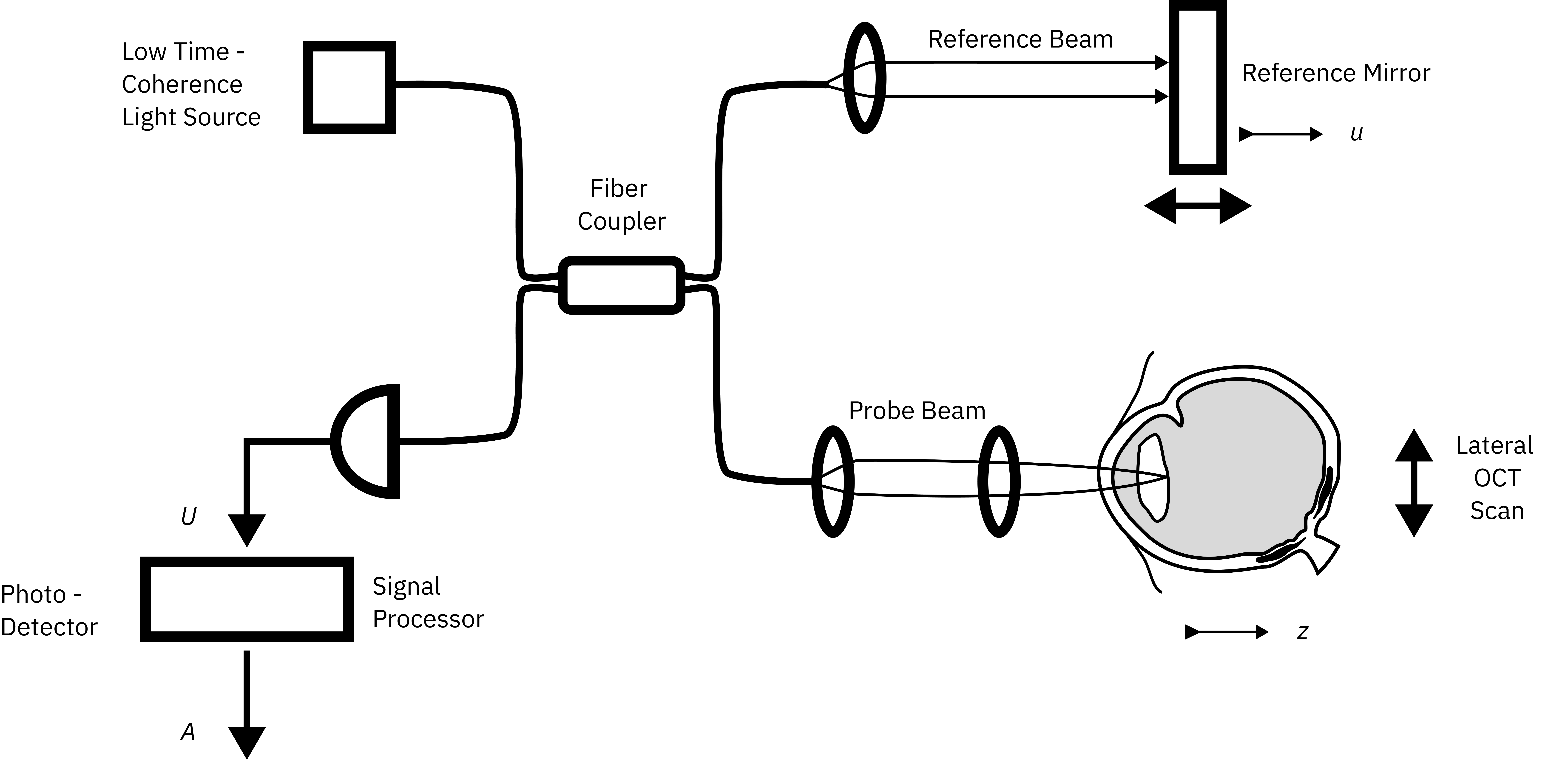
OCT Modalities
There are different modalities of OCT: Time Domain OCT (TD-OCT), Fourier Domain (FD-OCT), Spectral Domain OCT (SD-OCT) and Swept Source OCT (SS-OCT). Traditional OCT uses illumination sources in the IR range so that light travels faster through the tissues.
The first OCT modality is the TD-OCT, shown in Figure 1. The reference arm length is adjusted and the intensity resulting from the interference of the reflected light at the photodetector is used to obtain reflectance profiles of the sample. To overcome the need to physically move the reference arm, and obtain spectral information of the sample, Fourier Domain or Spectral Domain OCT were created. In this second approach, a spectrometer is placed instead of the TD-OCT detector, leading to the obtention of the spectral pattern of the reflected light after the interference, which is then used to obtain an A-Scan of the sample by means of its Fourier transform [1].
Furthermore, a third modality called Swept-Source OCT consists of sweeping the sample each time at a different narrow bandwidth wavelength and detecting each wavelength of the spectral interferometric signal consecutively by a fast single sensor [2].
The lateral resolution of all these modalities is determined by the characteristics of the light source; a low-coherence laser beam with a small beam diameter, large focus and broad bandwidth in the NIR is the ideal instrumentation for OCT.
In addition, there is also a rising interest in the use of Visible light to create 3D in vivo images with OCT (Vis-OCT). Several researchers are also exploring the use of this part of the spectrum to improve the axial resolution and to enhance the absorption and scattering produced by the tissue [2].
Iceblink Supercontinuum Laser for OCT
The Iceblink supercontinuum laser has demonstrated its versatility and outstanding performance in OCT measurements, which can be explained by several reasons:
- Broadest bandwidth that covers a large range in the NIR: 750 to 2300 nm.
- Low temporal coherence, thus long spatial coherence length, necessary for the interference between the reference and the probe beam.
- The Iceblink laser reduces the speckle of the OCT images, hence improving their resolution and getting a better SNR.
- Smooth and flattened spectral distribution to efficiently give information about the spectral characteristics of the sample.
- High optical power density, with more than 3 W of total power that goes deep into the sample without making any damage.
- High repetition rate of 80 MHz, which allows for the ultrafast illumination of the tissues. With the appropriate set of photodetectors, fast imaging speeds are achieved.